NEW CONSTRAINTS ON COSMOLOGY AND GRAVITY USING COSMIC VOIDS
An international team composed of three researchers from the CNRS and a professor from the UPMC, have put new constraints on the theory of general relativity – Einstein's theory of gravitation – using cosmic voids. These objects are regions of the Universe where the matter density is low; the space is thus nearly empty. The large number of the voids in the Universe makes them unique tools to probe the physics of gravity at low values of the matter density. By making use of the galaxy catalogs obtained from the Sloan Digital Sky Survey III, the researchers have again confirmed the predictions of the theory of general relativity. Their findings are published in the Physical Review Letters, dated August 25th 2016.
The cosmic voids: underused major components of the volume of the Universe
In the Universe, matter is spatially organized into four types of structures: clusters, walls, filaments and cosmic voids (see Figure 1), which globally form the cosmic web. The voids occupy nearly 75% of the volume of the Universe. Their content, both dark and bright, is concentrated predominantly on their edges. The rest of the matter held in the central part of these voids is attracted towards the borders, which leads to a decrease in the void matter content with time. Galaxies, which are the luminous component of matter, follow the large-scale structure of matter, and thus also delineate the voids. They are therefore used for measuring the matter density profile of the void borders.
Figure 1: Illustration of the large-scale structures of a virtual universe obtained by an N-body simulation, which allows to track the dynamical evolution of a set of particles under gravitational attraction. Color traces the matter density. The voids are thus clearly visible as black and dark areas. They are separated by walls and filaments (in blue and green), at the intersection of the latter lie clusters of galaxies (in yellow and red); these correspond to regions where the matter density is the highest. Credits: N. Hamaus/Ludwig Maximilian Universität München.
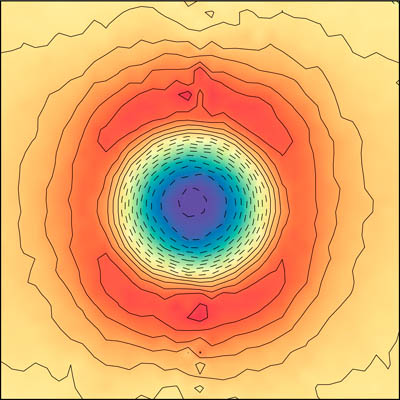
Credits: N. Hamaus/Ludwig Maximilian Universität München.
Cosmic voids are attractive “laboratories” to study the laws of physics governing gravity. Researchers of the collaboration have developed new analysis tools to make use of these particular regions of the Universe. These techniques rely on the measurement of the total observed velocity of galaxies lying on the borders of the voids (see Figure 2). This velocity is caused by two physical phenomena. A first component is due to the expansion velocity of the Universe which depends on the distance of the considered void from the observer (also called Alcock-Paczyński effect). The other component comes from the motions induced by the material content of the cosmic void itself, that is, mainly, the expulsion of matter towards the edge of the void (Kaiser effect).
New constraints on the theory of gravity
The researchers have applied this technique to the galaxies of the spectroscopic sample of the Sloan Digital Sky Survey III (http://www.sdss3.org), a deep sky survey of more than one million of galaxies in the northern hemisphere. The most distant galaxies are at 6 billion light-years from the Milky Way. The catalog provides for each galaxy its position on the sky, as well as the redshift, resulting from the two aforementioned velocity components. By carefully measuring the average characteristics of the cosmic voids identified using galaxies, the researchers have been able to put constraints on the cosmological parameters (governing the evolution of the Universe) and on the strength of the coupling between matter and the gravitational field. These measurements allow, for example, the derivation of constraints on the universal gravitational constant. Of course, many other aspects of the theory of general relativity are at play in leading to the actually measured values from the observations.
These constraints go beyond the existing ones in cosmology, while remaining complementary. They give access to information on the physics of the Universe at scales of a few tenths of millions of light-years. The other indicators of the gravitational field in cosmology are based on scales of either billions of light-years (e.g. the cosmic microwave background), or only of a few thousands of light-years (in galaxies).
Additionally, this new method allows one to test the theory of gravity in the low density environment of the interior of the cosmic voids. For example, some extensions of the theory of the general relativity (such as the so-called “f(R)” model) may predict higher velocities than expected from the classical theory for galaxies embedded in the same matter density field. In this case, the black contour lines of Figure 2 would have a different shape.
These results confirm the success of general relativity to explain observations. Future deep surveys of galaxies such as that performed by the Euclid mission (http://www.euclid-ec.org), will allow researchers to derive unprecedented constraints on the theory of relativity thanks to cosmic voids, notably through the 50 millions of galaxies that will be observed and the 10 times larger volume than in the SDSS survey.
This research has been supported by the IAP, the Labex ILP (ANR-10-LABX-63), the ANR (ANR-10-CEXC-004-01, ANR-11-JS56-003-01), and the NSF (NSF AST 09-08693).
Writing and contact
- Guilhem Lavaux
Institut d’astrophysique de Paris, CNRS, Sorbonne Université
guilhem [dot] lavaux [at] iap [dot] fr
Contacts
- Nico Hamaus
Ludwig Maximilian Universität München (former postdoctoral researcher at the IAP)
hamaus [at] usm [dot] lmu [dot] de
- Alice Pisani
Centre de Physique des Particules de Marseille, CNRS (former PhD student at the IAP)
pisani [at] cppm [dot] in2p3 [dot] fr
- Stéphanie Escoffier
Centre de Physique des Particules de Marseille (CPPM), CNRS
escoffier [at] cppm [dot] in2p3 [dot] fr
- Benjamin Wandelt
Institut d’astrophysique de Paris, CNRS, Sorbonne Université
benjamin [dot] wandelt [at] iap [dot] fr
- Paul M. Sutter
Ohio State University (ancien postdoctorant à l’IAP)
sutter [dot] 84 [at] osu [dot] edu
- Jochen Weller
Ludwig Maximilian Universität München
jochen [dot] weller [at] usm [dot] lmu [dot] de
Layout: Jean Mouette
September 2016